Introduction to Earthquakes
Earthquakes are natural phenomena that have fascinated and frightened humans for centuries. These sudden and violent shaking of the Earth’s surface can cause widespread destruction and loss of life. Understanding the occurrence and impact of earthquakes is crucial for communities living in earthquake-prone regions and for scientists studying the Earth’s dynamic processes. This article aims to provide a comprehensive overview of earthquakes, including their causes, types, measurement, frequency, distribution, impact, and strategies for mitigating their hazards.
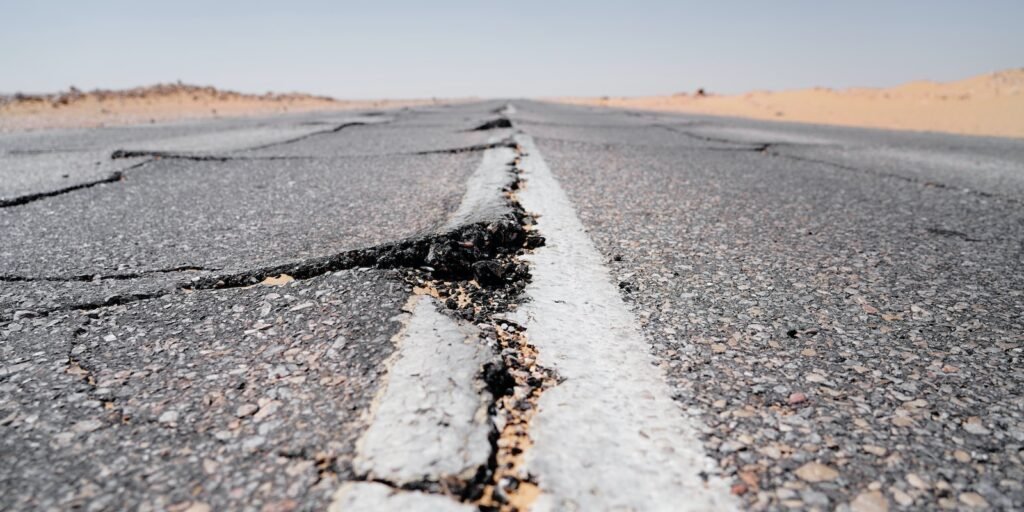
Plate Tectonics and Faults
To comprehend earthquakes, it is essential to understand plate tectonics, the theory that explains how the Earth’s lithosphere is divided into several large plates constantly moving relative to each other. Most earthquakes occur along the boundaries of these tectonic plates, where they interact. These interactions can result in the formation of faults, fractures in the Earth’s crust where rocks on either side have moved relative to each other. The three main types of faults are strike-slip faults, normal faults, and reverse faults, each contributing to different types of earthquakes.
Plate tectonics and faults are essential components of Earth’s dynamic puzzle, shaping the landscapes we see today. By understanding these geological processes, scientists are able to gain insights into how our planet has evolved over millions of years. This comprehensive overview aims to provide a clear understanding of plate tectonics and faults, highlighting their significance in Earth’s structure and the impact they have on our daily lives.
Plate tectonics is the scientific theory that describes the large-scale movement of Earth’s lithosphere, which is composed of several rigid tectonic plates. These plates float atop the semi-fluid asthenosphere layer that lies beneath them. The movement of these plates is driven by the convective flow of the underlying mantle, caused by heat generated from the Earth’s core. The lithosphere is broken into several major plates, such as the Pacific Plate, Eurasian Plate, and African Plate, along with numerous smaller plates.
The interaction of these tectonic plates is responsible for the formation of various geological features, such as mountains, valleys, and oceanic trenches. There are three main types of plate boundaries: divergent, convergent, and transform boundaries. At divergent boundaries, such as the Mid-Atlantic Ridge, plates move apart, allowing magma to rise from the mantle and create new crust. Convergent boundaries, such as the collision between the Indian and Eurasian plates that formed the Himalayas, involve the collision or subduction of plates, resulting in the formation of mountain ranges or volcanic activity. Transform boundaries, like the San Andreas Fault in California, occur when plates slide past each other horizontally.
Faults are fractures in the Earth’s crust where rocks on either side have moved relative to each other. They are the result of the immense forces acting upon the lithosphere, causing it to crack and deform. Faults can be classified into three main types: normal, reverse, and strike-slip faults. A normal fault occurs when tensional forces cause one side of the fault to move downward relative to the other side. These faults are typically found in areas of crustal extension, such as rift zones. In contrast, reverse faults occur when compressional forces cause one side of the fault to move upward relative to the other side. These faults are usually associated with convergent plate boundaries and the formation of mountains. Finally, strike-slip faults occur when horizontal shear forces cause rocks on either side of the fault to slide horizontally past each other.
Faults play a crucial role in redistributing stress within the Earth’s crust. When stress accumulates along the fault line, it can eventually be released in the form of an earthquake. The study of faults allows scientists to understand the behavior of earthquakes and predict areas at risk. Additionally, faults can also act as conduits for the movement of fluids, such as water or hydrocarbons, leading to the formation of valuable mineral deposits or important water reservoirs.
Plate tectonics and faults are fundamental concepts in the field of geology, providing a framework for understanding the dynamic nature of our planet. By studying plate tectonics, scientists can gain insights into the formation and destruction of continents, the distribution of natural resources, and the occurrence of natural disasters such as earthquakes and volcanic eruptions. Understanding faults helps us identify areas of high seismic activity and assess the potential risks associated with them. With ongoing research and technological advancements, our understanding of plate tectonics and faults continues to expand, contributing to the better understanding and management of Earth’s geological processes.
Causes of Earthquakes
Earthquakes can have various causes, but the vast majority are a result of the release of accumulated stress along faults. This stress builds up over time as tectonic plates push against each other or get stuck due to friction. When the stress becomes too great, it overcomes the friction, causing the rocks to slip suddenly, creating an earthquake. Other causes include volcanic activity, where magma movement can trigger seismic events, and human-induced earthquakes, such as those resulting from mining, reservoir-induced seismicity, or hydraulic fracturing.
Earthquakes have always been a fascinating and mysterious natural phenomenon. These powerful and often destructive movements of the Earth’s crust have captivated the curiosity of scientists and the general public alike. Understanding the factors that trigger earthquakes is crucial for predicting and mitigating their potential hazards. In this article, we will take a deeper dive into the root causes of earthquakes, exploring the intricate factors that play a significant role in their occurrence.
Earthquakes occur due to the sudden release of energy in the Earth’s crust, causing seismic waves that shake the ground. The primary cause of earthquakes is the movement of tectonic plates, which make up the Earth’s surface. These large sections of the Earth’s crust float and move on the semi-fluid mantle beneath. When two plates interact, they can either collide, slide past each other, or separate. These interactions create immense stress along the plate boundaries, which eventually becomes too intense, leading to an earthquake.
Another significant factor contributing to earthquakes is fault lines. Faults are fractures in the Earth’s crust where movements occur. When the accumulated stress along a fault line exceeds its strength, it snaps, releasing a tremendous amount of energy in the form of an earthquake. Fault lines can be classified into three types: strike-slip faults, where plates slide past each other horizontally; normal faults, where plates pull apart; and reverse faults, where plates compress against each other. Each type of fault exhibits distinct characteristics and contributes differently to the occurrence of earthquakes.
Volcanic activity is yet another factor involved in triggering earthquakes. In areas with active volcanoes, the movement of magma beneath the Earth’s surface exerts pressure on the surrounding rocks and triggers earthquakes. These volcanic earthquakes can occur before, during, or after volcanic eruptions. Additionally, the interaction between tectonic plates and the presence of magma chambers can also cause volcanic earthquakes. Understanding the relationship between volcanic activity and earthquakes is crucial, as it helps scientists predict volcanic eruptions and assess their associated risks.
While tectonic plate movement, fault lines, and volcanic activity play significant roles in causing earthquakes, there are several other factors that contribute to their occurrence. One such factor is the presence of fluids in the Earth’s crust, such as groundwater or oil. The movement of these fluids can alter the stress distribution within rocks, making them more susceptible to fracturing and triggering earthquakes.
Human activities, such as mining, reservoir-induced seismicity, or hydraulic fracking, can also induce earthquakes. These activities alter the stress distribution within the Earth’s crust, leading to the weakening of faults and triggering seismic events. Although human-induced earthquakes are typically much smaller than natural ones, they still pose risks, particularly in areas with significant human intervention.
Furthermore, the magnitude and intensity of earthquakes can be influenced by local geological conditions. The composition, density, and structure of rocks in an area can affect the propagation and amplification of seismic waves. Soft sediment layers, for example, tend to amplify ground shaking, potentially causing more significant damage to buildings and infrastructure.
Earthquakes are complex phenomena influenced by various factors. Understanding these factors is crucial for developing effective strategies to mitigate earthquake hazards. By studying tectonic plate movement, fault lines, volcanic activity, fluid presence, human activities, and local geological conditions, scientists can improve earthquake prediction and develop strategies to minimize their impacts. Continued research in this field will undoubtedly provide further insights into the intricate mechanisms behind earthquakes, ultimately leading to a safer and more resilient society.
Seismic Waves: Types and Characteristics
During an earthquake, energy is released in the form of seismic waves, which travel through the Earth. There are three main types of seismic waves: primary waves (P-waves), secondary waves (S-waves), and surface waves. P-waves are the fastest and can travel through solids, liquids, and gases. S-waves are slower but can only travel through solids. Surface waves, the most destructive type, move along the Earth’s surface and cause the shaking that we typically associate with earthquakes.
Seismic waves are the vibrations that occur in the Earth as a result of the release of energy during an earthquake. These waves play a crucial role in understanding the dynamics of our planet’s structure and the processes occurring beneath its surface. By studying seismic waves, scientists can gather valuable information about the properties of the Earth’s interior, monitor and predict earthquakes, and even explore other celestial bodies. In this article, we will delve into the types and key features of seismic waves, providing a comprehensive overview of this fascinating phenomenon.
Seismic waves can be classified into three main types: P-waves (primary waves), S-waves (secondary waves), and surface waves. Each type of wave exhibits distinct characteristics and travels through the Earth in different ways. P-waves are compressional waves that can travel through solids, liquids, and gases, making them the fastest seismic waves. S-waves, on the other hand, are transverse waves that cannot travel through liquids, as they require the shear strength of solid materials to propagate. Surface waves, as the name suggests, propagate along the Earth’s surface and are responsible for most of the destructive effects during an earthquake.
P-waves, also known as primary waves, are the first seismic waves to be detected during an earthquake. These waves compress and expand the materials they travel through, causing them to vibrate parallel to the direction of wave propagation. P-waves have the highest velocity among seismic waves, reaching speeds of up to 14 kilometers per second in the Earth’s crust. Due to their ability to travel through all mediums, including solids, liquids, and gases, P-waves are also the first waves to be felt by humans during an earthquake. However, their energy is relatively low compared to other types of waves.
S-waves, or secondary waves, are the second type of seismic waves to be detected. These waves cause particles to move perpendicular to the direction of wave propagation, creating a shearing effect. Unlike P-waves, S-waves cannot travel through liquids, such as the molten outer core of the Earth. They are slower than P-waves, typically traveling at speeds of around 8 kilometers per second. Due to their shear motion, S-waves are responsible for the majority of the shaking felt during an earthquake. This shaking can cause significant damage to buildings and structures, making S-waves of great concern in earthquake-prone areas.
Surface waves are the third type of seismic waves and are the slowest among the three. These waves travel along the Earth’s surface instead of passing through its interior. Surface waves are responsible for much of the destruction caused by earthquakes. There are two main types of surface waves: Love waves and Rayleigh waves. Love waves move side-to-side and cause horizontal shaking, similar to the motion of a snake slithering on the ground. Rayleigh waves, on the other hand, produce both vertical and horizontal motion, creating a rolling effect. The combination of these two types of surface waves can cause extensive damage to structures, making them crucial to understand when studying earthquakes.
Seismic waves are vital in understanding the nature and significance of earthquakes. By studying the types and key features of these waves, scientists can gain valuable insights into the Earth’s interior and monitor seismic activity. The different types of seismic waves, including P-waves, S-waves, and surface waves, exhibit distinct characteristics and travel through the Earth in various ways, contributing to the overall complexity of earthquakes. With ongoing advancements in technology and research, our understanding of seismic waves continues to deepen, allowing us to better comprehend and mitigate the devastating effects of earthquakes.
Measuring Earthquakes: Magnitude and Intensity
Scientists use two primary measures to quantify earthquakes: magnitude and intensity. Magnitude refers to the energy released at the earthquake’s source, which is determined using seismographs and measured on a logarithmic scale called the Richter scale. Intensity, on the other hand, measures the level of shaking experienced at a specific location and is described using the Modified Mercalli Intensity scale, which ranges from I (not felt) to XII (total destruction).
Earthquake Occurrence: Frequency and Distribution
Earthquakes occur worldwide, but they are not evenly distributed. Most earthquakes occur along plate boundaries, such as the Pacific Ring of Fire, which encircles the Pacific Ocean. This is due to the high tectonic activity in these regions. However, earthquakes can also occur within plates, known as intraplate earthquakes, although they are less frequent. The frequency and distribution of earthquakes are influenced by the interaction of tectonic plates and the geological history of a given region.
Earthquakes are natural phenomena that occur when there is a sudden release of energy in the Earth’s crust. They can cause significant damage and loss of life, making it crucial to understand their frequency and distribution. By studying these factors, scientists can better predict and prepare for future earthquakes. This article aims to provide a closer look at the frequency of earthquakes and how they are distributed around the world.
Earthquakes occur frequently around the globe, but their frequency varies depending on the region. Seismic activity is measured using the Richter scale, which determines the magnitude of an earthquake. The scale ranges from 0 to 10, with each increase in magnitude indicating a tenfold increase in energy release. While minor earthquakes with magnitudes below 3 are extremely frequent and often go unnoticed, those with magnitudes above 7 are rare but can cause significant devastation.
The frequency of earthquakes is not evenly distributed across the Earth’s surface. The majority of seismic activity occurs along tectonic plate boundaries. These boundaries are where the Earth’s lithosphere, which is divided into several large and small pieces called plates, interact with each other. The collision, subduction, or sliding of these plates can result in earthquakes. Therefore, regions with active plate boundaries, such as the Pacific Ring of Fire and the Alpine-Himalayan belt, experience a higher frequency of earthquakes. Conversely, areas located in the middle of tectonic plates, known as intraplate regions, usually experience fewer earthquakes.
Understanding the distribution of earthquakes is essential for assessing the level of seismic hazard in a particular area. Scientists use various methods to map earthquake distribution, such as analyzing historical earthquake records, studying fault lines, and using data from seismograph networks. These methods allow them to identify regions with high seismic activity and potential earthquake-prone areas.
One notable example of earthquake distribution is the Pacific Ring of Fire. This area encircles the Pacific Ocean and is characterized by intense seismic activity. Approximately 90% of the world’s earthquakes occur along the Ring of Fire, which is home to numerous active volcanoes and tectonic plate boundaries. Another region with a significant concentration of earthquakes is the Alpine-Himalayan belt, stretching from New Zealand to the Mediterranean Sea and Southeast Asia. By mapping earthquake distribution, scientists can focus their efforts on understanding the underlying causes and potential risks associated with specific areas.
Understanding the frequency and distribution of earthquakes is crucial for both scientific research and disaster preparedness. By analyzing seismic activity and mapping earthquake-prone areas, scientists can work towards better prediction and mitigation strategies. Additionally, this knowledge enables individuals and communities to take appropriate precautions and implement measures to minimize the impact of earthquakes. With continued research and advancements in technology, we can strive to minimize the devastating effects of earthquakes and create safer environments for those living in earthquake-prone regions.
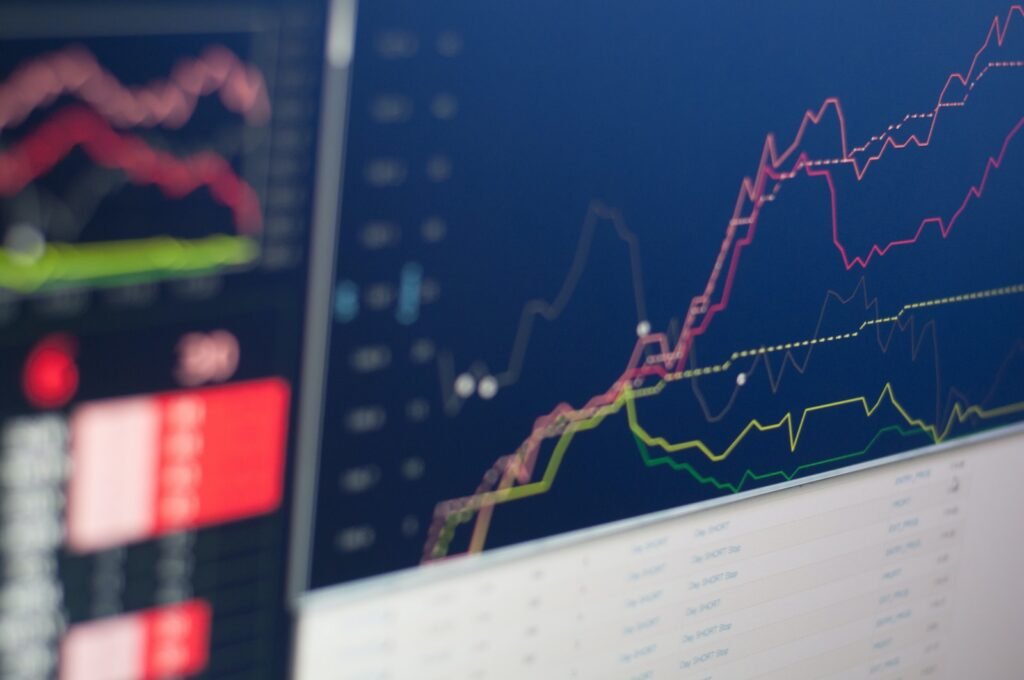
The Richter Scale: Understanding Magnitude
The Richter scale is the most commonly known scale for measuring earthquake magnitude. Developed by Charles F. Richter in the 1930s, it provides a logarithmic representation of the energy released during an earthquake. Each whole number increase on the Richter scale represents a tenfold increase in the amplitude of the seismic waves and approximately 31.6 times more energy released. While the Richter scale is widely recognized, scientists also use other scales, such as the moment magnitude scale (Mw), which provides a more accurate measurement for larger earthquakes.
Earthquake Impact: Human and Environmental
The impact of earthquakes can be devastating, causing the loss of human lives, injuries, displacement, and significant damage to infrastructure. The severity of the impact depends on various factors, including the magnitude of the earthquake, the distance from its epicenter, population density, building construction standards, and preparedness measures in place. Earthquakes can also have environmental consequences, such as landslides, tsunamis, and the release of harmful gases from the Earth’s interior.
Response and Preparedness: Building Resilience
In light of the destructive power of earthquakes, it is crucial for communities to be prepared and resilient. Early warning systems, public education, and emergency response plans are essential components of earthquake preparedness. Constructing buildings and infrastructure that can withstand seismic forces, implementing land-use planning regulations, and ensuring that critical facilities, such as hospitals and schools, are earthquake-resistant, are vital for minimizing the impact of earthquakes on human lives and infrastructure.
Mitigating Earthquake Hazards: Strategies and Challenges
Mitigating earthquake hazards involves a combination of strategies aimed at reducing vulnerabilities and risks. These strategies include enforcing building codes and regulations, retrofitting existing structures, and conducting seismic hazard assessments to inform urban planning decisions. However, implementing these measures can be challenging due to financial constraints, lack of awareness, and existing infrastructure that may not meet safety standards. Developing countries, in particular, face significant challenges in implementing effective mitigation strategies.
Earthquakes are natural disasters that can cause significant damage to infrastructure, loss of lives, and long-term economic repercussions. To mitigate the risks associated with earthquakes, various approaches have been developed and implemented. However, there are also several obstacles that need to be overcome to effectively reduce earthquake risks. In this article, we will explore the approaches and obstacles in reducing earthquake risks.
One of the key approaches in reducing earthquake risks is the implementation of strict building codes and regulations. These codes specify construction techniques and materials that can withstand seismic forces. By adhering to these codes, the structural integrity of buildings can be enhanced, reducing the likelihood of collapse during an earthquake. Additionally, retrofitting older buildings to meet the updated codes can also help minimize the risks. Regular inspections and enforcement of the codes are crucial to ensure compliance and improve the overall resilience of communities.
Public Awareness and Education
Another approach to reduce earthquake risks is through public awareness and education campaigns. These initiatives aim to inform and educate individuals about earthquake preparedness, response plans, and safety measures. By disseminating information about the potential risks and the necessary actions to take before, during, and after an earthquake, communities can be better equipped to handle such disasters. Public awareness campaigns can include public service announcements, educational programs in schools, and community drills to practice emergency procedures. Increasing public knowledge and understanding can significantly reduce the vulnerability of individuals and communities to earthquake hazards.
Early Warning Systems
Advancements in technology have led to the development of early warning systems for earthquakes. These systems use a network of sensors to detect the initial waves of seismic activity and quickly transmit warnings to potentially affected areas. Early warning systems can provide crucial seconds to minutes of advanced notice, allowing people to take immediate protective actions and enabling critical infrastructure, such as transportation systems and utilities, to initiate automated shutdown procedures. By providing timely warnings, these systems can save lives and reduce the impact of earthquakes on communities.
Lack of Resources
One of the main obstacles in reducing earthquake risks is the lack of resources. Implementing and enforcing building codes, retrofitting existing structures, and maintaining early warning systems require significant financial investments. Many regions, especially those with limited resources, struggle to allocate sufficient funds towards these initiatives. Additionally, ongoing maintenance and regular updates to building codes and warning systems are necessary to ensure their effectiveness. Overcoming the obstacle of limited resources requires a commitment from governments, international organizations, and communities to prioritize earthquake risk reduction and allocate appropriate funding.
Political will is another obstacle that often hinders efforts to reduce earthquake risks. Decision-makers may prioritize other issues over earthquake preparedness, especially in regions with infrequent seismic activity. Without strong political commitment and leadership, it becomes challenging to implement and enforce regulations, allocate resources, and promote public awareness campaigns. Overcoming this obstacle requires advocacy from scientists, experts, and communities affected by earthquakes to raise awareness and convince policymakers of the importance of investing in earthquake risk reduction.
Socioeconomic factors also pose obstacles in reducing earthquake risks. In many regions, poverty, informal settlements, and lack of access to resources contribute to increased vulnerability to earthquakes. These factors can lead to inadequate housing, weak infrastructure, and limited access to education and information. Addressing these socioeconomic factors requires a multi-faceted approach that includes poverty alleviation, urban planning, and community empowerment. By addressing the underlying issues, communities can become more resilient to earthquakes and other natural disasters.
Reducing earthquake risks requires a comprehensive approach that encompasses building codes and regulations, public awareness and education, and early warning systems. However, obstacles such as lack of resources, political will, and socioeconomic factors must be overcome to effectively implement these approaches. By addressing these challenges and working together, communities can strengthen their resilience and minimize the devastating impacts of earthquakes. It is essential that governments, organizations, and individuals prioritize earthquake risk reduction to create safer and more resilient communities.
Case Studies: Notable Earthquakes in History
Throughout history, numerous notable earthquakes have left lasting impacts on societies and shaped our understanding of these natural disasters. The 1906 San Francisco earthquake, the 1964 Great Alaska earthquake, and the 2011 Tohoku earthquake and tsunami in Japan are just a few examples. These case studies provide valuable insights into the causes, impacts, and responses to major earthquakes, helping scientists and communities learn from past experiences to enhance preparedness and resilience.
Conclusion: Advancing Earthquake Research
Earthquakes remain a complex and ongoing area of study, as scientists strive to improve our understanding of their occurrence, causes, and impacts. Through advancements in technology, such as improved seismological monitoring networks and computer simulations, researchers can gain valuable insights into the behavior of earthquakes and enhance our ability to predict and mitigate their hazards. By continuously advancing earthquake research, we can work towards minimizing the devastating consequences of these natural events and building safer and more resilient communities.